Gene Therapy Is Giving Us Incredible New Tools to Fight Disease. How Far Can It Go?
After decades of struggle, we’re finally seeing real-world, life-changing applications right here in Philadelphia. Has medicine finally got gene therapy right?
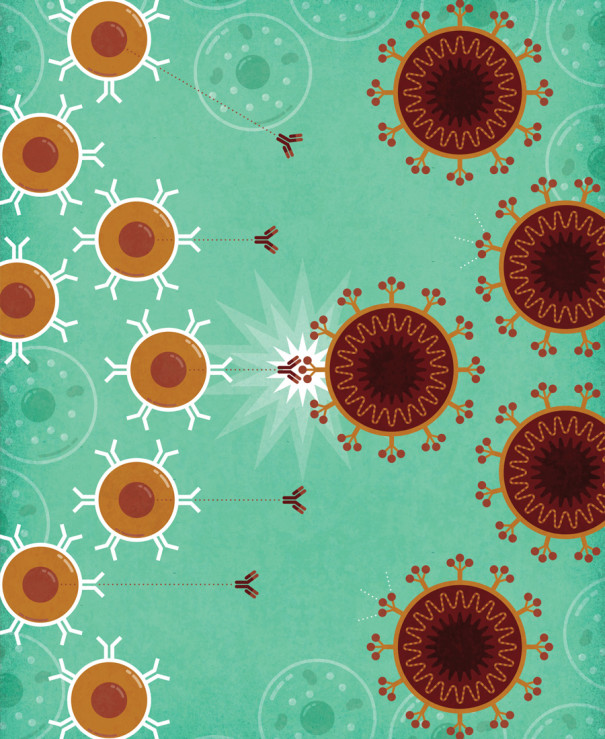
Below, learn how mRNA vaccines work to create coronavirus antibodies, plus how more gene therapy treatments are giving us options for combatting disease. Illustration by Jude Buffum
Think back to last spring. (You probably don’t want to, but humor me.) Those early months of the coronavirus pandemic in America weren’t all fear and chaos — though there certainly was that. There was also optimism, which, when one looks back on it now, seems completely untethered from reality.
You almost certainly heard it: Flu is worse. We are very, very ready for this. We’re just shutting down for two weeks. Considering our national death toll of more than half a million and counting, the optimism was clearly misplaced. Even so, one rosy prediction came true in a big way.
In March 2020, we were told a vaccine to prevent COVID-19 was a year to 18 months off — even though normal vaccine development takes years, often more than a decade, with no guarantee of success. We still don’t have vaccines to prevent AIDS or childhood RSV infections.
But on November 9th, pharmaceutical companies Pfizer and BioNTech announced that their two-dose vaccine was more than 90 percent effective in preventing COVID-19. Another company, Moderna, soon released similar news.
It turns out we had a lot going in our favor: a relatively easy-to-target virus, a gush of money, faster regulatory approval — and, for those first two vaccines, a breakout technology.
Both the Pfizer-BioNTech and Moderna vaccines use a molecular relative of DNA, called RNA, to train the body to fight off the coronavirus. These vaccines belong to a larger cadre of treatments that employ DNA, which makes up genes, and RNA, which in cells is derived from DNA. Both molecules contain instructions for the production of proteins, which cells need for nearly everything they do. Problems with proteins, such as the inability to manufacture a certain type, can lead to disease.
Some gene therapies work by supplying cells with new genetic material to compensate for disease-causing errors in genes, often by replacing missing proteins. Others endow cells with the ability to produce an entirely new protein. The vaccines, for example, give our cells the ability to briefly make a key piece of the virus so the immune system can learn to attack it.
Scientists have long sought to use genetic material as medicine, but doing so successfully has taken time and tears. Once-promising therapies have proven ineffective or impractical; some caused dangerous, even fatal, complications in patients who tested them. Clinical trials starting in the late 1990s, for example, led to cases of leukemia in some children treated for a rare immune condition and to the death of an 18-year-old, who suffered a massive inflammatory response to another therapy.
In recent years, however, researchers appear to have worked out some of the major issues by, for example, changing how genetic material is delivered to cells. Nowadays, a number of gene therapies are official tools of American medical care.
“Having lived through a couple of decades of hype and hope and then realism, I think that for the first time, there have been major advances in the gene therapy space that show us it can work,” says Beverly Davidson, a neuroscientist and gene therapy research faculty member at Children’s Hospital of Philadelphia and the University of Pennsylvania. About 30 years ago, she began exploring gene therapy as a means to treat degenerative neurological conditions, such as Huntington’s disease.
When effective, the emerging class of gene therapies can improve and even save lives. These treatments are already giving new hope to some cancer patients and relief to those suffering from certain debilitating genetic conditions. But with progress come new challenges. Doctors have had to learn to manage the potentially dangerous side effects of the cancer treatment, for example. And even if a gene therapy appears to have successfully treated a disorder, it’s often unclear how long the relief will last.
Researchers, meanwhile, continue to tinker with these therapies in hopes of improving them and adapting them to other diseases, raising the question: How far can gene therapy go?
— I —
The Future Is Now
Gene therapy took on a pandemic. What’s next?
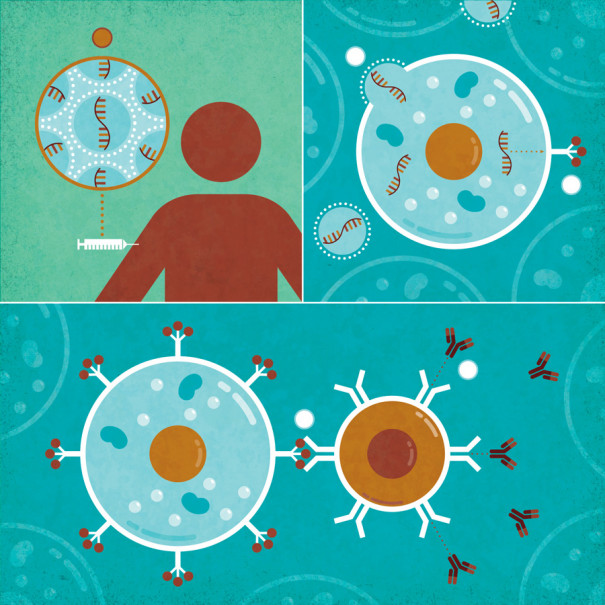
UNDERSTANDING GENE THERAPY: How COVID-19 mRNA Vaccines Work: 1) mRNA, packaged inside fatty spheres, enters the body. 2) The packages deliver the mRNA into cells, 3) which use it to make a protein from the coronavirus. 4) The immune system learns to recognize the protein and 5) produces Y-shaped antibodies that can fight the real virus. | Illustration by Jude Buffum
The field of gene therapy has deep roots in the biotech industry and hospitals here in Philadelphia. The mRNA COVID-19 vaccines are no exception.
In 1985, a Hungarian scientist named Katalin Karikó arrived at Temple University. At the time, she hoped to use RNA to treat viral infections, but an experimental attempt to treat HIV proved unsuccessful. In the years that followed, she struggled to maintain support for her research.
Meanwhile, scientists elsewhere had preliminary success injecting rodents with a specific variety of RNA, called mRNA — a molecule transcribed from DNA that the cell uses to make protein. This molecule, however, had drawbacks. In large enough amounts, for instance, it could provoke dangerous levels of inflammation.
A few years later, Karikó landed at Penn. In 1998, she met Drew Weissman, a physician-scientist who wanted to make an HIV vaccine. By tweaking one of the four letters comprising mRNA’s genetic code, the two researchers found, they could reduce the extreme immune response. What’s more, the altered mRNA had a much more potent effect on the activity of cells. “It was unbelievable, the dream come true,” Karikó says.
This discovery opened the door to a new category of vaccines as well as other therapies. By the time the pandemic began, efforts were already well under way to develop mRNA for use in vaccines to protect against infectious diseases. BioNTech, the German company where Karikó is now a senior vice president, had partnered with Pfizer to develop a vaccine for flu, an effort that became the basis for the company’s coronavirus vaccine. It was an easy swap. Conventional vaccines require the actual virus or pieces of it to teach the immune system to recognize and fight the real thing. However, the mRNA vaccines don’t use the virus at all, just the genetic instructions to make a small piece of it, so researchers can easily alter that code to tell the cells to make part of a new virus. Afterward, the mRNA is packaged up in fatty spheres that ferry it through the body and into cells.
Roughly nine months after COVID-19 formally became a pandemic, vaccinations began in the U.S. On December 18, 2020, Karikó and Weissman received their COVID-19 shots together, in an event organized by Penn to encourage trust in the vaccines.
Today, researchers and biotech companies, including BioNTech, are exploring ways to use mRNA, both with and without the one-letter tweak, to treat or prevent a horde of familiar threats: HIV, cancer, heart failure, genetic disorders, even autoimmune diseases. There are so many different applications, Karikó says, that “this is beyond even my imagination.”
— II —
Already Changing Lives
CAR-T therapies are evolving cancer treatment options.
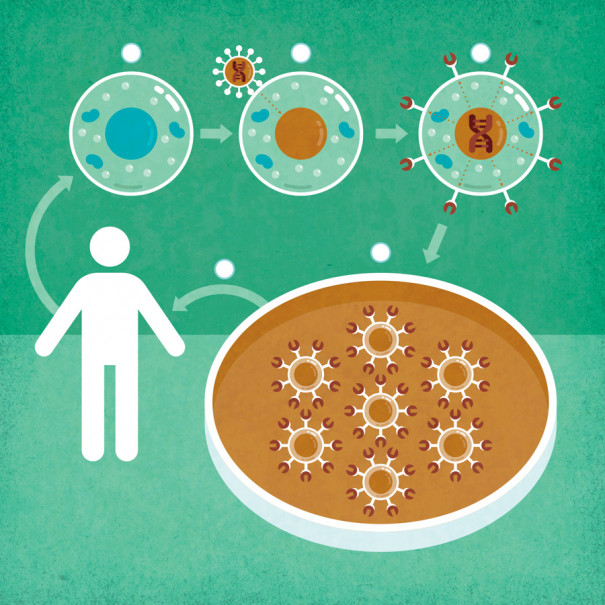
UNDERSTANDING GENE THERAPY: How CAR-T Fights Blood Cancer:
1) Immune cells called T cells are removed from the patient. 2) Empty shells of viruses deliver copies of a new gene to the T cells. 3) The gene instructs the cells to make a protein, called a CAR, designed to kill cancer cells. 4) The altered T cells are replicated, then 5) returned to the patient. | Illustration by Jude Buffum
The mRNA vaccines aren’t the only COVID shots to take a genetic approach. Others, including those developed by Johnson & Johnson and Oxford-AstraZeneca, as well as Russia’s Sputnik V, deliver genetic instructions in the form of DNA to make part of the coronavirus. Taken together, COVID-19 vaccines will touch millions, if not billions, of lives.
By comparison, most gene therapies now on the market are more boutique than big-box, offering relief to people with specific conditions. One of these, a new class of gene-based cancer treatments called CAR-T, has given oncologists a new way to beat back previously intractable cases of blood cancer.
In 2016, Jeff Tracy went to the doctor with a lump the size of half an egg on the left side of his neck. He had chronic leukemia. At first, his cancer — malignant B cells, once a normal part of his immune system — grew relatively slowly. But in time, his indolent cancer turned aggressive, presenting a more immediate threat to his life. He received a bone marrow transplant, but the cancer returned. “Anytime you relapse after a bone marrow transplant, the prognosis is very, very poor,” says David L. Porter, a hematologist-oncologist at Penn who treated Tracy. A quick relapse was even worse.
Tracy’s wife, Penny, was with her husband when Porter gave them the news that the transplant appeared to have failed. The doctor said he wanted a week to think about what to do next.
After the couple left his office, they followed their standard post-appointment ritual. “When we got to the elevator, we would give each other a kiss and say it was a good day,” Penny says. “Even when we found out the bone marrow transplant didn’t work, we still gave each other a kiss and said, ‘It’s a good day; he’s thinking about us.’ Because as long as Porter is thinking about us, that means we have hope.”
A week later, Porter recommended a new treatment called CAR-T. And this is where fortune cut Jeff Tracy a break.
The previous year, in 2017, the FDA had approved the first two CAR-T therapies, giving doctors permission to use them for specific types of difficult-to-treat blood cancer. Tracy’s chronic leukemia had developed into one of them, large B-cell lymphoma, and the timing of his relapse in the spring of 2018 meant he could receive the CAR-T treatment called Yescarta.
The drug would be Tracy’s own T cells, another type of immune cell, harvested from his blood and, like characters from a comic book, genetically altered to possess an unnatural power: to find and destroy their former comrades, the now-malignant B cells.
Tracy welcomed this option. “I wasn’t afraid. I was anxious to get it started,” he says.
First, he had to temporarily give up his T cells. While he lay in a hospital bed, a centrifuge separated them from his blood, the rest of which was returned through an intravenous line. The cells were sent across the country to a manufacturing facility in California, where they received the genetic instructions to make an artificial protein known as a chimeric antigen receptor, or CAR. Scientists had designed this protein to latch onto both healthy and cancerous B cells. With its guidance, Tracy’s altered T cells would destroy his B cells.
A few weeks later, on August 21st, Tracy got his cells back intravenously. The most difficult part came next.
The newly empowered T cells can instigate an intense flu-like illness. For Tracy, this manifested as a fever that spiked as high as 104 degrees Fahrenheit. He did escape the more mysterious neurological side effects, which can include confusion, difficulty speaking and writing, and even seizures.
Nearly three years out, his cancer is still gone. Not only have the malignant B cells disappeared; some healthy ones have returned. He does, however, suffer from graft-vs.-host disease, an immunological condition caused by his unsuccessful bone marrow transplant. It has left him with mouth sores, dry eyes, and tight skin that hinders his movements.
“Is it a pain in the butt? At times, yes, but you know what? I’m aboveground, so I don’t care,” says Tracy, now 62.
When CAR-T works, it can be dramatically effective for adult lymphoma, Porter says. “But it doesn’t work in everybody,” he adds. “And while many patients respond, only about 40 percent have long-term, ongoing responses. Jeff is one of those people.”
Doctors want to increase those odds as well as offer the therapy to more of their patients. Right now, they have permission to treat blood cancers with CAR-T only in patients who have relapsed or fail to respond to conventional options, like chemotherapy or a bone marrow transplant.
“Potentially, CAR-T can completely change how we treat patients,” says Henry Fung, a Fox Chase Cancer Center hematologist-oncologist. Like Porter, he hopes to see the therapy offered to patients earlier in the course of their illnesses.
“In the future, we may be able to prevent relapse,” he says. “Then we may not need transplants anymore, and we may not need chemotherapy anymore.”
Already, as doctors have figured out how to manage the potentially dangerous side effects, they’ve been able to offer the treatment to more people. “When we first started,” says Fung, “I was very reluctant to do it for anyone older than 55 years old. But now, I don’t have any problem with 70-year-olds.”
He sees one mundane — but major — drawback to CAR-T: accessibility. As a new, highly specialized treatment, CAR-T can only be administered at medical centers accredited to perform bone marrow transplants. Patients who live in remote rural locations can face logistical difficulties accessing these centers, which are often in urban areas, at major hospitals.
Preparing the cells also requires a lot of time — a precious commodity for cancer patients. During the three-to-four-week wait to get their cells back, patients can see their diseases worsen — or they can die, Fung says. He’s now involved in clinical trials for a potential solution: pre-made CAR-T cells, available on demand. “If it works, there will be another major revolution,” he says.
While doctors anticipate treating more blood cancers this way, there are also efforts under way to see if genetically engineered T cells can effectively treat solid cancers, like those of the lung or pancreas.
— III —
Clearing the Hurdles
New therapies for hemophilia are closing in on the finish line.
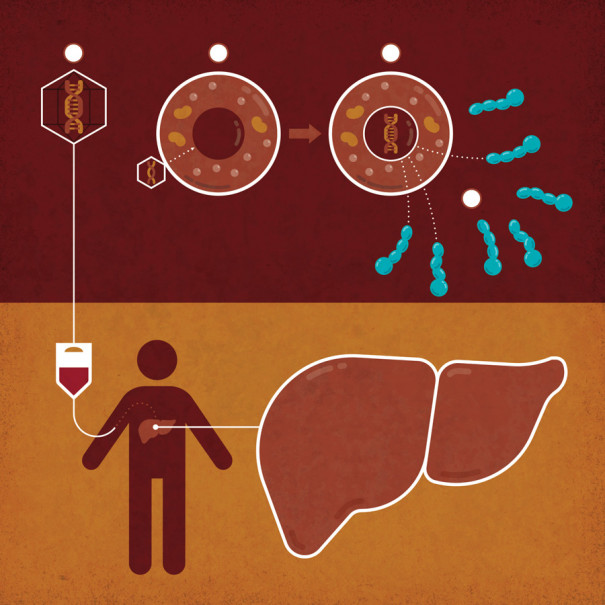
UNDERSTANDING GENE THERAPY: How Hemophilia Treatments Compensate for Defective Genes: 1) A gene for a missing blood-clotting protein is ferried to the liver within the empty shells of viruses, which 2) deliver the gene to the liver cells. 3) The gene directs the production of the protein, 4) which is secreted into the blood. | Illustration by Jude Buffum
Cancer isn’t the only disease for which doctors expect to gain new options relatively soon. The FDA anticipates approving a growing number of gene therapies. New treatments for hemophilia, a blood-clotting disorder, may be among them.
In April of 2017, Bill Maurits, who has hemophilia B — one of two common forms of this disorder — received one of these experimental therapies at CHOP through an intravenous line in his left arm.
Because of a genetic mutation, Maurits, 51, doesn’t have enough of the numerous clotting factors that, in domino-like fashion, collaborate to cause blood to coagulate. Without this clotting factor, a protein called factor IX, Maurits’s clots “are like a brick wall with no mortar,” he says. “They’re there, but they don’t have any strength or holding power.”
As a teenager, Maurits played full-contact sports and jumped BMX bikes — all the things other kids in his neighborhood did but his doctor discouraged. The impacts against pavement and other kids didn’t cause him to gush blood, but they did exact a toll. Blood leaked into his joints, inflicting pain, swelling and long-term damage. While the bleeding required numerous transfusions in his early childhood, eventually treatment evolved, and he started getting injections of clotting factor IX instead.
That meant a needle inserted into a vein in his left arm, which eventually became so scarred that he could no longer feel the punctures. When Maurits read about a clinical trial for a gene therapy, he saw a way out. “If nothing else, I could reduce the number of infusions and number of times I had to stick myself,” he says. “Just that by itself would be a plus. Because no matter what any hemophiliac says, no matter how easy they say it is to stick themselves, sticking yourself sucks.”
The first clinical trial testing a gene therapy for hemophilia began in 1998. At the time, the disease seemed like low-hanging fruit. Not only does it have a simple genetic cause; a treatment wouldn’t need to be 100 percent effective, since a person can get by with lower-than-normal levels of the missing factor.
However, attempts to develop gene therapies for hemophilia, as for other diseases, have faced formidable technical challenges. First off, the new genes must get into the right places, and cells there must use them to produce enough of the protein a person lacks to actually matter.
“If you don’t make enough of the protein, there’s nothing else to talk about, right? You need to go over that hurdle first,” says Denise Sabatino, a gene therapy researcher at CHOP and Penn who works on hemophilia.
To make gene therapies safer and more effective, scientists have looked into improving the delivery method, among other things. The new genes are often ferried into cells inside the empty shells of viruses, which can’t cause infection. Over the past 20 years, researchers have developed new viral shuttles as well as a virus-free method — the one employed by the mRNA vaccines.
The gene therapy that caught Maurits’s attention, which is now Pfizer’s Benegene, uses an updated viral shuttle to deliver the gene. After a conversation with Lindsey George, a hematologist and director of clinical in vivo gene therapy at CHOP and the lead investigator on the trial, Maurits felt comfortable signing up. “They’ve come a long way, to the point where there really wasn’t, as far as I saw, a whole lot of risk involved,” he says.
The infusion of new genes took about an hour, and within a week or so, it started to kick in. Maurits’s factor IX levels remain below normal but have increased enough that he’s nearly stopped injecting it, getting it only for surgeries, including one to replace a knee destroyed by bleeding. Physical activity still makes his damaged joints sore, but he bounces back much more quickly. “It was life-changing, honestly,” he says.
Of the 15 participants, Maurits is the only one to bleed since receiving the therapy, George says. So far, no major safety concerns have emerged. “It’s clearly liberating for them. They’re able to just kind of wake up and live their lives,” George says. “I find it humbling that a one-hour intravenous infusion of a medication can end up making this long-lasting, remarkable improvement in people’s lives.”
For Maurits, the improvement he’s experienced feels almost like a cure. Researchers aren’t ready for that word.
“At this moment in time, we don’t really know how long the expression will last after gene therapy,” Sabatino says. “We hope it will last for the lifetime of the patient. But we don’t actually know yet.”
— IV —
On the Horizon
Untangling genes’ roles in heart disease in the quest for new therapies
As diseases go, hemophilia makes for a comparatively amenable target. The country’s most prolific killer, heart disease, is another story. This organ can malfunction in many ways and for any number of reasons. Over the past few decades, a number of drugs, devices and procedures have been developed to keep the heart pumping.
Gene therapy isn’t among them, but that’s not for lack of trying. Researchers and biotech companies are actively working on treatments for heart problems.
One prospective therapy in development in Philadelphia has roots in a tragedy. In 1983, a 23-year-old named Lisa Berger died at Johns Hopkins University Hospital in Baltimore after being diagnosed with congestive heart failure. Doctors told the family that it was probably a coxsackievirus infection — typically the cause of hand, foot and mouth disease in children — that had damaged her heart.
“I think everyone in the family just assumed she had a weak heart,” says Dana Weidman, Berger’s cousin. But in the years that followed, one of Weidman’s brothers suffered heart failure, too, and she became suspicious.
“I started saying, ‘There’s no way they have a virus,’” says Weidman, now 57. A filmmaker who teaches at a New York State community college, she documented her search for answers in an independent film, Nobody’s Perfect.
In it, she describes how the family suffers from a condition formally known as dilated cardiomyopathy. It occurs when the heart muscle weakens and stretches like a worn-out elastic waistband, potentially to the point where it can’t pump enough blood. The condition often arises as a result of coronary artery disease, but sometimes, the muscle dilates for no obvious reason. Over the years, researchers have attributed a growing portion of these mysterious cases to genetic mutations.
In 2003, a cardiologist at Thomas Jefferson University Hospital connected the Weidmans to Arthur Feldman, a cardiologist who studies the genetic causes of heart disease. Feldman gathered blood samples from family members willing to provide them, but it would be another decade before he and some colleagues could pin down the mutation: a small section missing from the DNA within a gene known as BAG3.
As the team was preparing to publish this discovery in 2014, Feldman requested Berger’s medical records. Among them, he spotted his own signature and realized that decades before, he had cared for her while he was a medical resident at Johns Hopkins. “Sadly, we really didn’t have anything to offer at the time,” he says. “It was so devastating when she died.”
It was then that he resolved to further study BAG3, with the goal of helping patients like Berger. In the years that followed, he and his colleagues tested a preliminary form of gene therapy in mice with BAG3 mutations. By delivering working copies of BAG3, they found, they could improve the rodents’ hearts’ ability to pump blood.
Feldman, now at Temple University, has since founded a biotech company, Renovacor, to develop this therapy, which is still being tested in animals. While some evidence suggests that raising BAG3 levels could help heart patients without mutations, Renovacor is focused on developing a therapy specifically for the roughly 50,000 Americans in whom defects in BAG3 produce dilated cardiomyopathy. If successful, such a therapy would bring new precision to the treatment of at least a tiny portion of heart patients.
Scientists believe that genetics contribute to most diseases, at least to some degree. However, finding ways to tailor patients’ care to their genetic vulnerabilities — through gene therapies or by other means — has proven challenging.
It turns out genes and disease have a complex relationship. Take BAG3: It’s one of dozens of genes linked to dilated cardiomyopathy. Different mutations in BAG3 can produce different disorders, including neurological ones. And, of course, many cases of dilated cardiomyopathy don’t have a clear genetic cause. Weidman and her mother, Dottie, now 82, appear to have benefited from such vagaries. Both carry the family’s mutation but have led largely healthy lives.
Feldman, however, is optimistic, noting that the pace with which research is teasing out these connections has accelerated enormously since he was introduced to Weidman’s family nearly 20 years ago. Now, for instance, it takes just days for a commercial lab to deliver a readout of the section of a person’s genetic code that contains BAG3.
“We’re kind of beginning that journey,” Feldman says of attempts to uncover the genetic underpinnings of disease. “The world has opened up to us.”
Editing Out Disease?
Gene-editing technology CRISPR-Cas9 is giving researchers even more options.
Over time, researchers have devised a number of ways to manipulate the genetic activity within cells, often by giving them new DNA or RNA instructions to follow.
A gene-editing technology, known as CRISPR-Cas9, could make it possible to finesse these changes by revising a part of the genetic code a cell already possesses — akin to rewriting the original directions, rather than adding new instructions alongside them.
CRISPR, the subject of the 2020 Nobel Prize in Chemistry, gives researchers not only more precise control but also more options, says Stephan Grupp, a pediatric oncologist at CHOP. “You can do a lot more than just put a gene in, although putting a gene in is a pretty impressive thing to do all by itself.”
In experiments, for instance, it’s possible to direct CRISPR to the exact spot needed to fix a broken gene, add or turn one off, he says. The FDA has yet to approve any gene therapy using CRISPR, but clinical trials have begun testing a few of these experimental treatments in people. Grupp is involved in one such study focused on sickle cell disease, a painful genetic disorder resulting from a faulty version of the protein hemoglobin in red blood cells.
That treatment, which Vertex Pharmaceuticals and CRISPR Therapeutics are developing, switches off the production of abnormal hemoglobin and turns on a healthy type of the protein. CRISPR accomplishes this swap by changing a key gene in blood-forming cells while they’re outside the body; the altered cells are then returned to the patient.
At the beginning of the study, researchers hoped to reduce the episodes of acute pain that accompany sickle cell disease. Early results suggest they exceeded their goal: The episodes appear to have stopped entirely for all three patients who received the treatment.
In December, the first patient treated had been episode-free for nearly a year and a half, and so far there appears to be no lasting side effects. No one knows, however, how long the treatment will continue to be effective. “We hope it lasts forever,” Grupp says, “but we have no idea.”
Other studies have begun testing CRISPR-based gene therapy in people suffering from an inherited form of blindness and in cancer patients. Many more preliminary efforts are seeking to use gene editing to attack other illnesses, including one that has, for decades, eluded medical science’s ability to cure.
About 35 years ago, early in Temple University neurovirologist Kamel Khalili’s career in science, he remembers talking with his mentor, National Cancer Institute researcher George Khoury, about another deadly virus: human immunodeficiency virus, or HIV, which had recently been identified as the cause of a fatal immune-system-disabling syndrome.
At the time, scientists knew the virus didn’t just infect cells; it infiltrated their genetic code, inserting its own genes into the cell’s DNA, the entirety of which is known as the genome.
“We were just saying that the only way one can cure the disease is to eliminate the virus from the host genome,” says Khalili. “But we didn’t know how we could do it. We didn’t have a tool; we didn’t have the knowledge.”
The arrival of CRISPR offered Khalili the tool he needed. He and his colleagues adapted it to excise the viral code from the genomes of infected cells, most of which are part of the immune system. A biotech company Khalili cofounded, Excision BioTherapeutics, is now developing a gene therapy based on their research.
The company doesn’t yet have approval to start clinical trials in people, but preliminary studies with mice and monkeys have given Khalili hope that one day, a CRISPR-based gene therapy could accomplish a decades-old goal: curing HIV infection.
“I think it’s long, long overdue,” he says.
Published as “The War to End Disease” in the May 2021 issue of Philadelphia magazine.